Zeno of Citium › Achaean League » Ancient origins
Articles and Definitions › Contents
- Zeno of Citium › Who Was
- Achaean League › Antique Origins
Ancient civilizations › Historical and archaeological sites
Zeno of Citium › Who Was
Definition and Origins
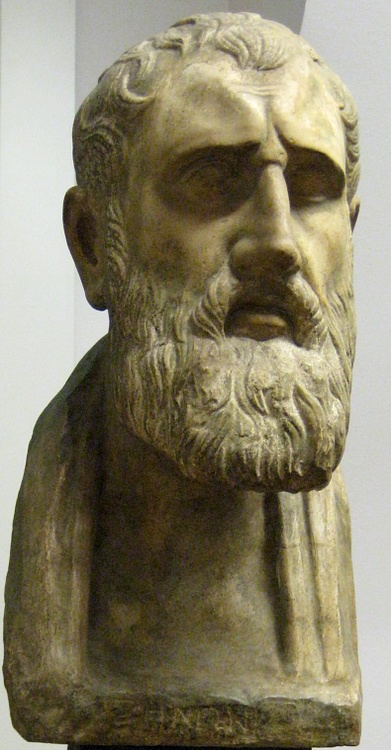
Zeno of Citium (c. 336 – 265 BCE) was the founder of the Stoic School of philosophy in Athens which taught that the Logos (Universal Reason) was the greatest good in life and living in accordance with reason was the purpose of human life. If one lived according to the instinct of impulse and passion, one was no more than an animal; if one lived in accordance with universal reason, one was truly a human being living a worthwhile existence. This philosophy would later be developed by Epictetus (50-130 CE) and others and would have a great impact on the people of Rome, most notably the emperor Marcus Aurelius (121-180 CE). Stoicism would eventually become one of the most popular and influential philosophies in the Romanworld. Zeno was born in the Phonecian- Greek city of Citium on Cyprus in the same year that Alexander the Greatascended to the throne of Macedonia. His father was a merchant who traveled often to Athens and Zeno, naturally, took up his father's profession. It is unclear whether Zeno studied philosophy in his youth but, around the age of 22, while stranded in Athens after a shipwreck, he picked up a copy of Xenophon ’s Memorabilia and was so impressed by the figure of Socratesthat he abandoned his former life and made the study of philosophy his only interest.
Zeno studied under Crates of Thebes and then under Stilpo the Megarian and then became the pupil of Polemo. From each of these men he learned some different aspect and nuance of the life of a philosopher. From Stilpo, for example, it is said he learned that the greatest fault in life lay in saying `yes' too quickly to any request and one should avoid doing so in order to live a tranquil life. In this, he pre-dates Sartre's assertion that saying `no' is an assertion of one's personal identity while agreeing to another's request diminishes the individual personality. After many years of study, Zeno set up his own school and began to teach on the porches (the `stoa') of the arcade in the market place in Athens and so his school took the name of the place of learning, Stoic.
ZENO BELIEVED ONE SHOULD COURT REASON AND RECOGNIZE THAT ALL THINGS ARE IMPERMANENT AND WITHOUT LASTING VALUE.
It is alleged that Zeno said, “I made a prosperous voyage when I was shipwrecked” and by this he meant that, prior to his coming to Athens, his life had no meaning. The discipline of philosophy gave Zeno a focus he seems to have lacked as a merchant and he devoted himself to study and, more importantly, to living the values he absorbed from his teachers and the books he read. Professor Forrest E. Baird writes that Zeno "argued that virtue, not pleasure, was the only good and that natural law, not the random swerving of atoms, was the key principle of the universe" (505). He was praised highly by the Athenians for his temperance, his consistency in living what he taught, and his good effect on the youth of the city. Zeno never seems to have been one to hold his tongue when he saw what he perceived as foolishness in the youths around him and many of his remarks sound similar in tone to statements Diogenes of Sinope would have made. Unlike the "mad Socrates" of the Agora (as Diogenes was known), Zeno lived a life of traditional, Athenian, respectability while refusing to compromise his principles for what society valued.
It was clear to Zeno that most of the people of Athens suffered because they desired what they did not have or feared losing what they loved. The pursuit of pleasure, as espoused by the Epicurean's philosophy (which sprang from the Cyrenaic School of Aristippus) could never possibly satisfy a human being because one would always be chasing after what one desired or trying to hold on to what one had already obtained. Instead of pleasure, one should court reason and recognize that all things are impermanent and without lasting value. Once one understood this, one would achieve a state of enlightened apathy in which one would be set free from "enslavement to one's passions" (Mautner, 607). This belief is what made the stoic school so popular to the Greeks of the time and, later, to the Romans: Zeno's teachings cleared the mind and allowed one to see beyond what one thinks one wants to recognize all that one actually needs - which is simply the self. If one is self-aware, one is also aware of others and, further, recognizes that it is in simplicity that true contentment may be found. These teachings, of course, are more well known today as the basic tenets of Buddhism.

Zeno of Citium
Diogenes Laertius, writing in the 3rd century CE, preserved some of Zeno's teachings in his work Lives and Opinions of Eminent Philosophers. He writes that Zeno claimed:
As for the assertion made by some people that pleasure is the object to which the first impulse of animals is directed, it is shown by the Stoics to be false. For pleasure, if it is really felt, they declare to be a by-product, which never comes until nature by itself has sought and found the means suitable to the animal's existence or constitution; it is an aftermath comparable to the condition of animals thriving and plants in full bloom. And nature, they say, made no difference originally between plants and animals, for she regulates the life of plants too, in their case without impulse and sensation, just as also certain processes go on of a vegetative kind in us.But when in the case of animals impulse has been superadded, whereby they are enabled to go in quest of their proper aliment, for them, say the stoics, Nature's rule is to follow the direction of impulse. But when reason by way of a more perfect leadership has been bestowed on the beings we call rational, for them life according to reason rightly becomes the natural life. For reason supervenes to shape impulse scientifically (Baird, 507).
In this, Zeno is simply saying that animals pursue pleasure because they are governed by instinct which drives them to impulse; but human beings, since they have been given reason, ought to be governed by rational thought and live reasonably.To pursue pleasure as the meaning of life, and think that one is living well, is to be no more than an animal or, as Shakespeare later phrases it in Hamlet, "What is a man, if his chief good and market of his time be but to sleep and feed? A beast, no more. Sure he that made us with such large discourse, looking before and after, gave us not that capability and god-like reason to fust in us unused" (Act IV.iv.33-39). To be a true human being, one needed to behave like a true human being: rationally.
When he studied under Crates of Thebes, Zeno wrote his Republic which is quite a different vision of the perfect soceity than the ideal city state as imagined by Plato in his work of the same name. Zeno's Republic is a utopia whose citizens claim the universe as their home and where everyone lives in accordance with natural laws and rational understanding. Men and women were completely equal in society's eyes and there was no injustice because all actions proceeded from reason. There were no laws necessary because there was no crime and, because everyone's needs were taken care of in the same way that animals are in nature, there was no greed, nor covetousness nor hatred of any kind. Love governed all things and everyone living in this cosmopolis understood they had what they needed and wanted for nothing more. It is thought that this vision was largely inspired by Crates' life and that of his wife Hipparchia of Marneia who lived on completely equal terms with him, wore men's clothes, and taught philosophy to men. Crates and Hipparchia lived their lives in accordance with the simplicity of reason and Zeno's vision in his Republic reflects that view. Of Zeno's work, Plutarch later wrote:
It is true indeed that the so much admired Republic of Zeno, first author of the Stoic sect, aims singly at this, that neither in cities nor in towns we should live under laws distinct one from another, but that we should look upon all people in general to be our fellow-countryfolk and citizens, observing one manner of living and one kind of order, like a flock feeding together with equal right in one common pasture. This Zeno wrote, fancying to himself, as in a dream, a certain scheme of civil order, and the image of a philosophical commonwealth.
Zeno lived and taught in Athens from the time he arrived there following his shipwreck until his death. He died, apparently from suicide, after he tripped coming out of school and broke his toe. Lying on the ground, he quoted a line from the Niobe of Timotheus, “I come of my own accord; why call me thus?” and then, interpreting the accident as a sign he should depart, strangled himself. While this may seem a strange end to the life of a man who preached the primacy of reason, it would not have seemed so to him. When some happy period of one's life ends, it is irrational to cling to the past and wish it would return;nothing can make that time come again and longing for an impossible past only robs one of the present. Zeno was an old man when he is said to have broken his toe and, realizing that he had lived a good and meaningful life in Athens, he may have simply concluded that it was time for him to move on to something else.
Achaean League › Antique Origins
Definition and Origins

The Achaean League (or Achaian Confederacy) was a federation of Greek city -states in the north and central parts of the Peloponnese in the 3rd and 2nd centuries BCE. With a combined political representation and land army, the successful early years of the League would eventually bring it into conflict with other regional powers Sparta, Macedon, and then later Rome. Defeat by the latter in 146 BCE brought the confederacy to a dramatic end.
FOUNDING & MEMBERSHIP
The League was formed in c. 281 BCE by 12 city-states in the region of Achaea who considered themselves as having a common identity ( ethnos ). Indeed, several of these states had already been members of a federation ( koinon ) in the Classical period but this had broken up c. 324 BCE. The principal founding members of the League were, then, Dyme, Patrai, Pharai, and Tritaia, all located in western Achaea in the northern Peloponnese of Greece. More Achaean cities joined in the following decade and the stature of the League grew when Sicyon, a city outside the region, joined in 251 BCE. From then on, membership steadily grew to encompass the whole of the Peloponnese.
Members enjoyed the strength in numbers of the League whilst maintaining their independence. Their primary obligation was to contribute a quota of warriors for the League's collective army. Cities also sent representatives to meetings of the League in proportion to their status - smaller cities sent one, and larger ones could send three. Of these, the original founding and larger members continued to exert more influence and their representatives certainly carried more stature as regional statesmen.The representatives met, perhaps four times each year, on a federal council and there was also a citizen's assembly. Up to c.189 BCE meetings were held at the sanctuary of Zeus Homarios at Aigion and thereafter at individual city-states, presumably on a rotation basis.
THE LEAGUE GAVE ITS MEMBERS A BETTER DEFENCE AND BROUGHT SUCH BENEFITS AS ACCESS TO A COMMON JUDICIAL PROCESS AND A COMMON CURRENCY.
The representatives sent by city-states were led by the strategos (general), a position which was introduced in c. 255 BCE and held for one year. To better ensure one state did not overly dominate, the position could not be held for consecutive years.However, this did not stop some notable figures such as Philopoimen (from Megalopolis) and Aratos (from Sicyon) holding the position several times in their careers. Other important positions included the cavalry commander ( hipparch ), ten damiourgoiofficials, and a League secretary.
The League not only gave its members a better defence against outside aggression but also brought several non-military benefits such as access to a common judicial process and the use of a common currency and system of measurements.
SUCCESSES
As the League expanded and became more influential, so too its relations with other regional powers increased in intensity.Local rivalries existed in particular with Sparta to the south and the Aitolian League across the straits of Corinth. Even distant Macedon and Egypt began to take an interest in the League's affairs. These relations became ever more strained as the League became more ambitious. In 243 BCE Corinth was attacked and forcibly made a member of the League. The effect of this acquisition was to weaken the Macedonian presence in the region and so allowed the League to assume more member cities, notably Megalopolis in 235 BCE.
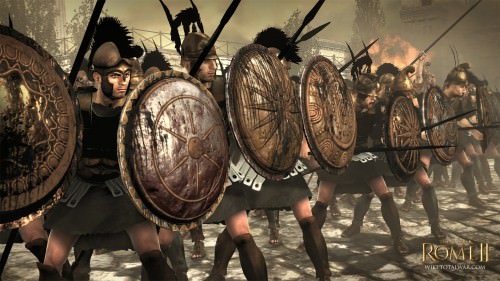
Greek Phalanx
THE MACEDONIAN WARS
Trouble was brewing, though, as Cleomenes III of Sparta (r. 235-222 BCE) sought to expand his own influence in the region.This forced the League to seek help from Antigonos III of Macedon. Together the two allies defeated Sparta at the Battle of Sellasia in 222 BCE. As payment for their support, the acropolis of Corinth, the Acrocorinth, was given back to the Macedonians.
Then a new heavyweight power entered the scene of Greek inter-state politics: Rome. The League remained loyal to Macedon in the First Macedonian War (212-205 BCE) between the two powers. This was an unwise move as Philip V's Macedonian army was defeated. The Achaeans then pragmatically switched sides in the Second Macedonian War (200-196 BCE) and supported Rome. This time finding itself on the winning side, the League had to carefully balance its ambitions with the new wider political situation. Around 196 BCE Rome and the League signed a treaty of alliance, quite a distinction at the time.

Achaean League c. 150 BCE
CONFLICT WITH ROME & COLLAPSE
Sparta, Elis, and Messene were made members of the League while Rome was distracted by another war, this time against Antiochos III, the Seleucid king. Again the Romans were unstoppable and their defeat of Antiochos at Thermopylae in 191 BCE and Magnesia in Asia Minor in 190 BCE left Greece ever more vulnerable to Roman dominance. A Third Macedonian War (171-167 BCE) brought another Roman victory and Greece was well on the road to become nothing more than a Roman province.
Already not best pleased by the League's acquisition of Sparta, Rome became suspicious of its ambiguous political stance. As a consequence, Rome took 1,000 prominent Achaean hostages back to the Eternal City and in 146 BCE there was open war between the two powers in what is sometimes referred to as the Achaean War. Predictably, the Roman war machine prevailed again; Corinth was sacked and the League in its current form disbanded. The confederacy was, though, later permitted to function in a more limited way and on a more local basis. It survived as such into the 3rd century CE and perhaps beyond, occasionally forming alliances with other such groups within the Greek region of the Roman Empire.
LICENSE:
Article based on information obtained from these sources:with permission from the Website Ancient History Encyclopedia
Content is available under License Creative Commons: Attribution-NonCommercial-ShareAlike 3.0 Unported. CC-BY-NC-SA License